Decoding Bacterial Resistance: How Our Medicines Are Being Outsmarted
Introduction
The Bacterial Cell In
A Nutshell
Bacteria are single-celled organisms, usually round (coccus), rod (bacillus), spiral or vibrio (comma-shaped). Knowing a little about the anatomy of bacteria is useful in understanding how antibiotics affect bacteria, allowing us to understand how they function, grow, and reproduce. It also can help us understand how bacteria can outsmart our first line of defence against infection. This is a simplified view and will assist in understanding the action of antibiotics and the ways bacteria can develop resistance to them.
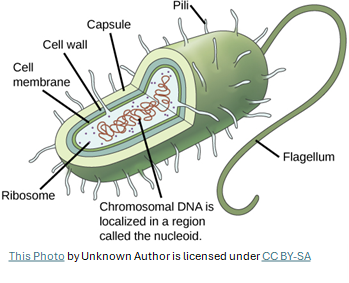
- Cell Wall: This protective outer layer surrounds the bacterium, providing structural support and preventing it from bursting. Antibiotics like penicillin target the production of the cell wall.
- Cell Membrane: Located beneath the cell wall, the cell membrane controls what enters and exits the cell
- Ribosomes: These are essential for protein synthesis, which is crucial for bacterial growth and survival. Antibiotics such as tetracyclines target ribosomes to disrupt the synthesis of proteins.
- DNA: Contains the genetic information necessary for bacterial function, reproduction, and stability. Another antibiotic, ((fluoro)quinolone), halts the uncoiling of the DNA, needed for reproduction and repair.
Some bacteria also have other components:
- Porins: Channels in the outer membrane allowing antibiotics to enter the cell.
- Efflux Pumps: Act like "tiny bouncers" that pump antibiotics out of the cell.
- Biofilms: Communities of bacteria encased in a slimy matrix, making it harder for antibiotics to penetrate the individual bacterial cell.
How Antibiotics Work
- Beta-lactams, like penicillin, inhibit the production of a crucial component of bacterial cell walls, causing the bacteria to burst.
- Tetracyclines, target ribosomes, which are essential for protein synthesis, disrupting bacterial growth and survival.
- Cell wall synthesis inhibitors: Block the formation of the protective cell wall, causing the bacteria to burst. Examples include beta-lactams (penicillin and cephalosporins) and glycopeptides (vancomycin).
- Cell membrane disruptors: Damage the cell membrane, leading to leakage of essential cell contents. For example, polymyxins (used in some multi-drug-resistant infections).
- Folate synthesis inhibitors: Prevent the production of folate, vital for bacterial growth. Examples given are sulphonamides (sulphur drugs).
- DNA gyrase inhibitors: Stop the uncoiling of DNA necessary for reproduction and repair. DNA is present in all cells but coiled. It is required to be “straightened out” before it can be used by the cell.
- RNA synthesis inhibitors: Block RNA production needed for protein synthesis, with examples like annamycin's (Clindamycin) and rifamycins.
- Protein synthesis inhibitors: Disrupt protein synthesis within the cell machinery essential for
growth. Examples include tetracyclines and macrolides.
A little understanding of how bacteria function allows the appreciation of how bacteria can develop resistance to our antibiotics.
Mechanism Of Antibiotics
Resistance
Efflux Pumps: These act like tiny bouncers in the bacterial cell membrane, actively pumping antibiotics out of the cell, preventing them from reaching their target.
1. Limiting Antibiotic Entry.
- Efflux Pumps: These act like tiny bouncers in the bacterial cell membrane, actively pumping antibiotics out of the cell, preventing them from reaching their target.
- Porin Changes: Some bacteria have channels (porins) in their outer membranes that allow antibiotics to enter. Changes in the number or structure of these porins can reduce the amount, therefore the dosage, of antibiotic taken into the cell.
- Biofilms: These are communities of bacteria encased in a slimy film making it
harder for antibiotics to penetrate the individual bacterial cell.
- Enzymatic Destruction: Bacteria produce enzymes that can break down antibiotics. A classic example is beta-lactamase, which inactivates penicillin-like drugs.
- Chemical Modification: Some bacteria can add extra groups to the chemical structure of antibiotics, preventing them from working as they should.
3. Modifying the Antibiotic Target.
- Target Mutation: Antibiotics bind to specific targets within bacteria. Mutations in the genes in these targets can change their shape, preventing the antibiotic from binding to it correctly.
- Target Bypass: Some bacteria can find alternative pathways or targets that the antibiotic doesn't affect, allowing them to continue with essential processes even in the presence of the antibiotic
- Reduced Metabolism: Some antibiotics work by disrupting the metabolism of the bacteria. Bacteria in a dormant or slow-growing state may be less susceptible to these particular antibiotics.
- Increased Production of Target: In some cases, bacteria can increase the production of the antibiotic's target, making it harder for the drug to bind to all of them and have a significant effect.
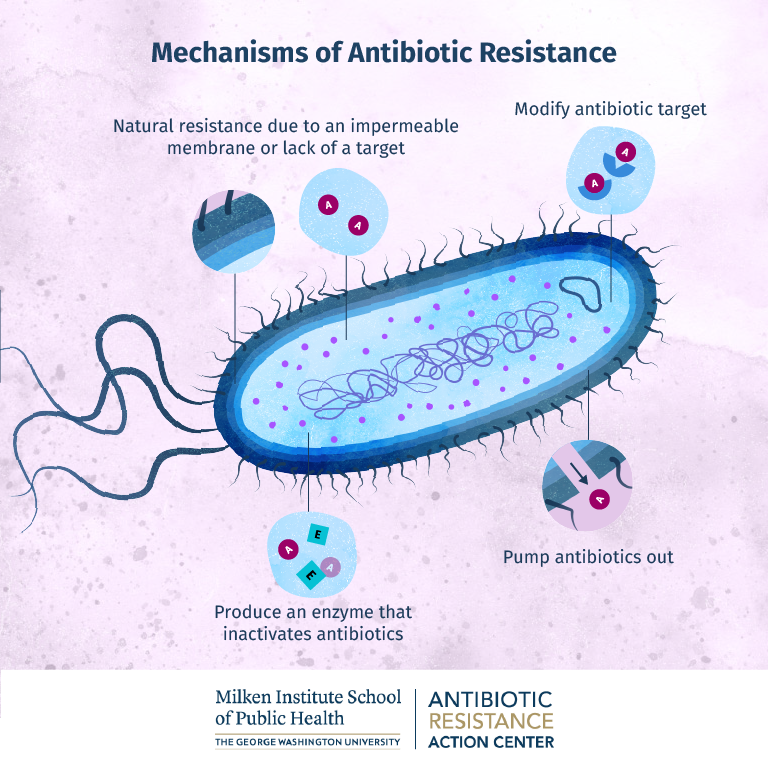
The Evolution And
Spread Of Resistance
Horizontal gene transfer allows bacteria to share resistance mechanisms, even between different species.
Antibiotics create selection pressure, favouring the survival and reproduction of resistant bacteria.
While resistance mechanisms can be costly to the bacterium, if the benefits of surviving antibiotics outweigh the costs, the resistant bacteria will dominate within a population.
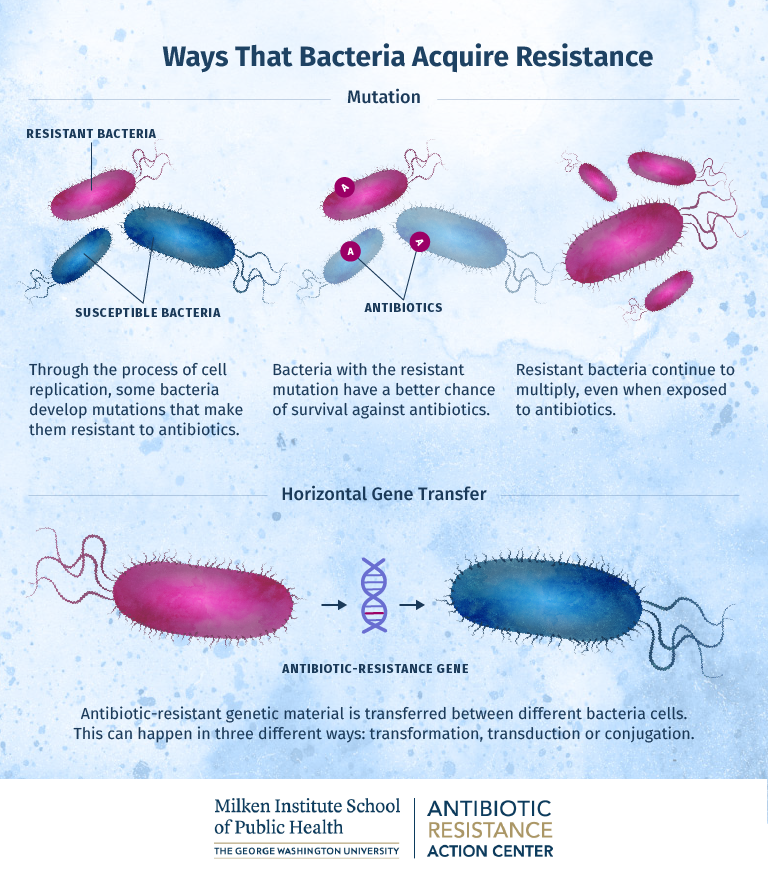
SUPERBUGS
- Methicillin-resistant Staphylococcus aureus (MRSA): is resistant to methicillin and related antibiotics, causing skin infections, pneumonia, and bloodstream infections.
- Carbapenem-resistant Enterobacterales (CRE): Resistant to carbapenems, a class of “last-resort antibiotics”, causing urinary tract infections and pneumonia.
- Multidrug-resistant Pseudomonas aeruginosa: Resistant to multiple antibiotics, causing pneumonia and bloodstream infections. It is especially dangerous in those with weakened immune systems.
- Drug-resistant Neisseria gonorrhoeae: This bacterium causes gonorrhoea, a sexually transmitted infection that is becoming increasingly difficult to treat due to antibiotic resistance.
FIGHTING BACK: WHAT CAN BE DONE?
Addressing antibiotic resistance requires a
multifaceted approach. Using antibiotics differently, using a combination of
antibiotics with different actions on the bacterial cell, or utilising the specific
actions some antibiotics have. Antibiotic stewardship promotes responsible
antibiotic use in human and animal health to slow down resistance development.
Science can therefore work towards developing new antibiotics, new ways of
using known antibiotics, or alternatives to these wonder drugs. What can we do:
- Combination Therapy: Combine existing antibiotics to overcome bacterial resistance
mechanisms.
- Resistance-Breakers: Develop drugs that specifically disable resistance mechanisms, such
as efflux pump inhibitors, to stop the bacterium from being able to pump
antibiotics out of its cell.
- Antimicrobial Peptides: Utilise naturally occurring substances with antibacterial activity
that are less prone to resistance development.
- Phage Therapy: Use viruses (bacteriophages) that specifically infect and kill
bacteria.
- Global Collaboration: Foster international cooperation to share knowledge, resources, and
develop effective strategies to fight resistance and develop new therapies.
- Rapid Diagnostics: Develop rapid diagnostic tests to quickly identify the cause of infections
and determine antibiotic susceptibility.
- Reviving Old Antibiotics: Some older antibiotics, for example, polymyxins, fell out of favour
due to toxicity concerns, but with modifications and new delivery methods, they
could be repurposed against resistant strains.
- CRISPR-Cas Systems: This gene-editing technology could be used to target and destroy
specific resistance genes in bacteria, making them susceptible to antibiotics
again.
- Infection Prevention: Implement effective infection control measures in aged care and other healthcare settings. By reducing the number of infections in a facility, we would reduce the need for antibiotics in the first place. AMS has shown us that the prolific use of antibiotics is often unnecessary and with better diagnoses we can treat infections more specifically.
CONCLUSION
Antibiotic resistance is a serious and growing threat, potentially leading to a post-antibiotic era in the not-too-distant future. Combating this challenge requires a concerted effort. By using antibiotics wisely, practising good hygiene, and supporting research, we can all play a role in preserving the effectiveness of these life-saving drugs. What steps can we take individually and collectively to ensure that antibiotics remain effective for future generations? Dive deeper into this critical topic by exploring our related articles or subscribing to our newsletter for the latest updates.
Lyndon Forrest
I am a passionate and visionary leader who has been working in the field of infection prevention and control in aged care for almost 30 years. I am one of the co-founders and the current Managing Director and CEO of Bug Control New Zealand and Australia, the premium provider of infection prevention and control services in aged care. I lead a team that is driven by a common purpose: to help aged care leaders and staff protect their residents from infections and create a healthier future for them.
I am building a business that focuses on our clients and solving their problems. We are focused on building a world-class service in aged care. We focus on being better, not bigger, which means anything we do is for our clients.
Erica Leadley
Erica Leadley is a dedicated professional with a rich background in agriculture and nutrient management. Growing up on her family's farm in Mid Canterbury, she developed a deep passion for farming. She currently resides on her partner's arable property in South Canterbury.
In 2017, Erica joined the Farm Sustainability team, focusing on nutrient management and environmental stewardship. In February 2024, she became the Manager of Marketing and Sales at Bug Control New Zealand - Infection Prevention Services, where her passion now includes improving infection prevention outcomes.
Outside of work, Erica loves cooking and traveling, often combining her culinary interests with her explorations in Italy and Vietnam. She enjoys entertaining family and friends and remains actively involved in farm activities, especially during harvest season.
Toni Sherriff
Toni is a Registered Nurse with extensive experience in Infection Prevention and Control. Her career began as a kitchen hand and caregiver in Aged Care facilities, followed by earning a Bachelor of Nursing.
Toni has significant experience, having worked in Brisbane’s Infectious Diseases ward before returning home to New Zealand, where she continued her career as a Clinical Nurse Specialist in Infection Prevention and Control within Te Whatu Ora (Health NZ).
Toni brings her expertise and dedication to our team, which is instrumental in providing top-tier infection prevention solutions to our clients.
Julie Hadfield
Julie is experienced in Accounts & Payroll Administration & after a long career in both the Financial & Local Government Sectors, is now working with our team. Julie brings her strong time management & organisational skills to our team, which is important to keep the company running in the background to enable the rest of our team to provide top notch service to all of our clients.
Andrea Murray
I attended Otago University in NZ and graduated as a Dental Surgeon. After 40 years in the profession, I retired in 2022. Infection prevention knowledge was part of everyday practice, dealing with sterilisation, hand hygiene, and cleaning.
Before retiring, I began doing some editing and proofreading for Bug Control as I am interested in the subject and in the English language. During the COVID-19 lockdown, I attended the ACIPC course "Introduction to Infection Prevention and Control", which increased my interest in the subject. I now work part-time as the Content Editor for the company.
Princess
Princess began her career as a dedicated Customer Service Representative, honing her communication and problem-solving skills. She later transitioned into a Literary Specialist role, where she developed a keen eye for detail. Her journey then led her to a Sales Specialist position, where she excelled in client relations.
Now, as a Customer Support professional in Infection Prevention Services. Princess focuses on ensuring customer satisfaction, building loyalty, and enhancing the overall customer journey.
Dianne Newey
With over 35 years of experience as a Registered Nurse, I'm now applying all my experience and skills as a Senior Infection Prevention and Control Consultant with Bug Control Infection Prevention Advisory Services.
This is through IP&C education, IP&C environmental audits and reports, IP&C policy and procedure review and development and consultancy on infection prevention and control issues. When I’m not working, I spend time with my family and in my garden, where I grow all my own veggies.
Caoimhe (Keva) Stewart
Caoimhe is the Manager of Customer Service at Bug Control | Infection Prevention Services, where she ensures that learners have a seamless and supportive experience. With her previous experience as a Registered Nurse in both the UK and Australia, Caoimhe brings a deep understanding of healthcare to her role. Before joining Bug Control IPS Services, she worked in a variety of nursing settings, including Occupational Health, Palliative Care, and Community Nursing, providing her with the ability to empathise with learners and understand the challenges they face.